Research
My main research interests are in cosmology and galaxy formation. I use computational simulations to study the physical processes that are important in the formation and evolution of galaxies. I am particularly interested in the role that the chemistry of interstellar gas plays. Chemistry is a critical aspect in understanding galaxy formation, as observations of galaxies often involve emission and absorption lines from ions and molecules, which trace different gas phases. By modelling the chemical processes that determine the abundances of these species, we can make predictions for these observables from our simulations that we can compare directly to observations. This allows us to test our models for galaxy formation, and helps us determine the physical properties of the gas probed by the observations. Accurate chemical modelling is also important for understanding how gas cools, as different species radiate away the thermal energy at different rates via transitions between excited states.
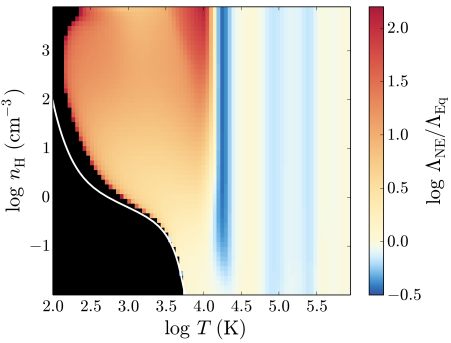
Figure showing the ratio of non-equilibrium to equilibrium cooling rates as a function of temperature and density, for gas that is cooling isochorically from 106 K. Richings et al. (2014)
Non-equilibrium chemistry
As gas in a galactic halo cools, it falls to the centre of the halo and fuels star formation in the galaxy. Gas cooling therefore plays an important role in galaxy formation. Cosmological simulations often compute cooling rates assuming chemical equilibrium, where the chemical reactions between ions and molecules have reached a steady state. However, this assumption of chemical equilibrium may not be valid if the physical conditions of the gas are evolving rapidly, for example in the presence of turbulence or a fluctuating UV radiation field. To investigate the effects of non-equilibrium chemistry, I have developed CHIMES, a chemical model that tracks the non-equilibrium evolution of ions and molecules in interstellar gas, which can be incorporated into hydrodynamic simulations of galaxies. In Richings et al. (2014), we used this model to show that non-equilibrium effects can enhance the cooling rate by up to two orders of magnitude in gas that is cooling rapidly (see the figure on the right).
Simulations of isolated galaxies
Movies of the gas evolution in three simulations of an isolated disc galaxy, with a metallicity of 0.01 Zsol (left), 0.1 Zsol (centre) and Zsol (right), looking at the disc of the galaxy face on (top row) and edge on (bottom row). Richings & Schaye (2016)
I have incorporated CHIMES into several hydrodynamics codes that are commonly used for cosmological simulations, including GADGET (last described in Springel 2005), AREPO (Springel 2010), GIZMO (Hopkins 2015), and SWIFT (Schaller et al. (2018)). Using GADGET and CHIMES, we ran a series of simulations of isolated disc galaxies with an initial stellar mass of ~109 Msol, with different metallicities and UV radiation fields (Richings & Schaye 2016). The movie on the left shows the evolution of gas in three of these simulations, with a fixed metallicity of 0.01 Zsol (left), 0.1 Zsol (centre) and Zsol (right). We see that, as the metallicity increases, there is much more star formation, and hence more stellar feedback, for example from supernovae. This trend of increasing star formation rate with metallicity occurs because metals are efficient coolants, so they cool the gas down to the cold (~100 K), star-forming phase. We also repeated these simulations using cooling rates that were computed assuming chemical equilibrium. We find that non-equilibrium chemistry has little impact on the global properties of the galaxy, such as the total star formation rate. However, it does affect the abundances of individual chemical species, particularly in molecular gas. For example, the CO emission from the galaxy changes by a factor ~2-4 when we include non-equilibrium effects.
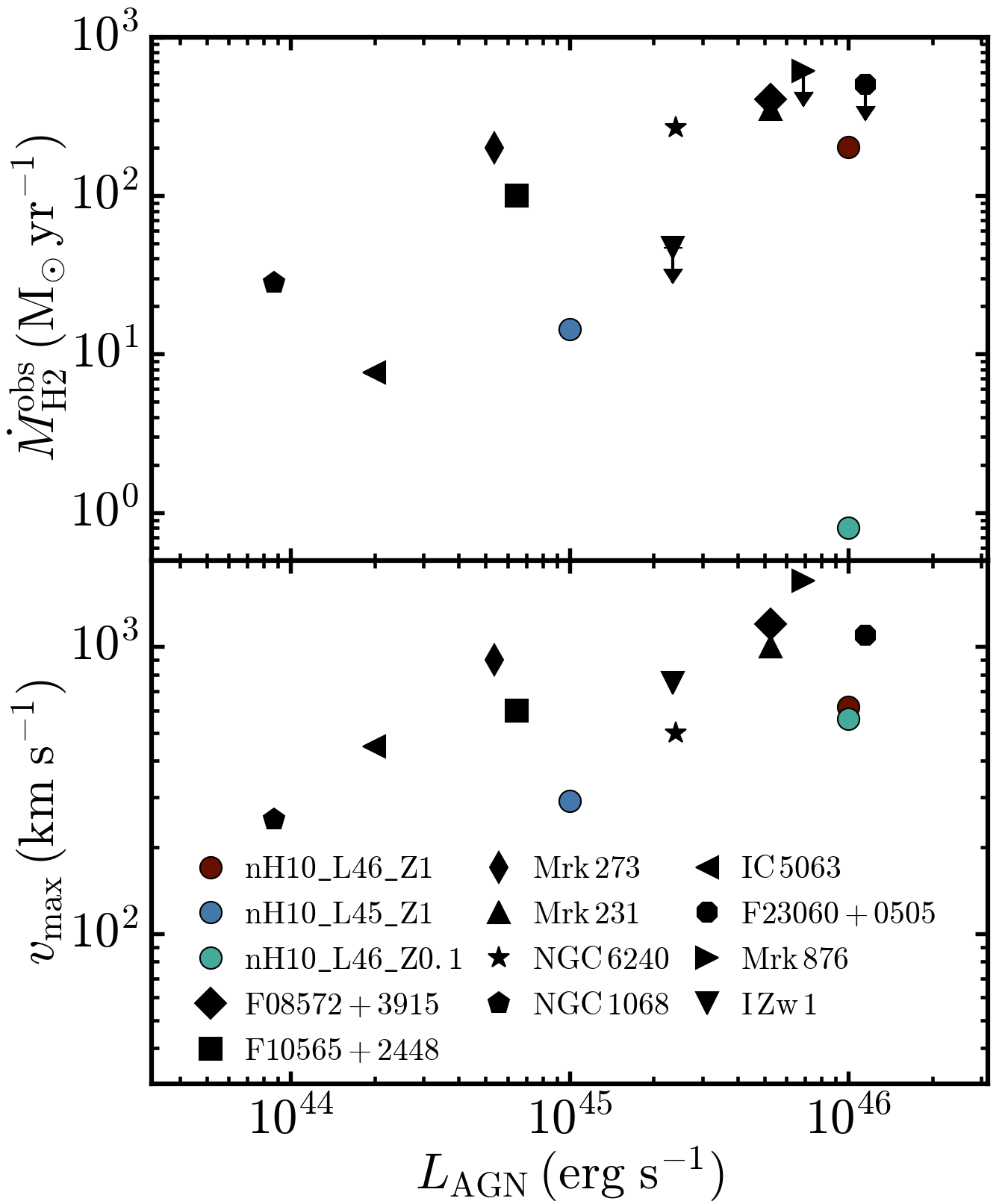
Molecule formation in AGN-driven winds
I have also applied CHIMES to idealised simulations of an isotropic AGN wind interacting with a uniform ambient medium (Richings & Faucher-Giguère 2018). The aim of this project was to understand the origin of the fast (~1000 km s-1) molecular outflows that have been observed in luminous quasars. Previous studies have looked at whether these outflows can be formed by sweeping up existing molecular clouds in the host galaxy. However, they found that the clouds tend to be destroyed by the hot, fast AGN wind before they can be accelerated to the high velocities seen in observed outflows. We therefore used a suite of simulations of an AGN wind, including the time-dependent chemistry of the molecules, to explore whether molecules can form in-situ, within the AGN wind.
We found that in-situ molecule formation can produce molecular outflows with outflow rates up to 140 Msol yr-1. For comparison, observations of AGN host galaxies have found molecular outflow rates up to 100 - 1000 Msol yr-1. The figure on the right shows the outflow rates (top panel) and maximum line of sight velocities (bottom panels) from our simulations (coloured circles) and from the observed AGN host galaxies from Cicone et al. (2014), plotted against AGN luminosity. For the simulations, we used the CO abundances calculated from the chemical model to create model CO spectra, which we used to compute the outflow rates in the same way as the observations, which are also based on CO spectra. We see that, for the two simulations at solar metallicity (red and blue circles), the outflow rates are close to, albeit slightly lower than, the observed outflow rates. However, the simulations all underpredict the observed velocities by a factor ~2.
Last modified: Fri Nov 4 13:57:35 GMT 2022